Redefining Single Photon Detection Technology: Vacuum Tube-Based Detector Solutions
Contents:
High-End Vacuum Tube Detector Based Solutions
Vacuum Tube Detector: Working Principle
Single Photon Counting with an MCP-PMT
Single Photon Imaging with an Image Intensifier Tube
Single Photon Imaging and Counting Using a TPX3 Chip
High-End Vacuum Tube Detector Based Solutions
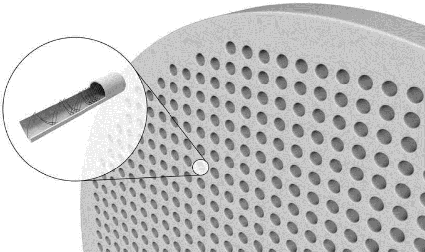
Photonis Single photon detection solutions are based on vacuum tube detector technology. This technology combines high detection efficiency (QE) with an extreme low amount of dark counts (dark noise). After pulsing, the phenomena in which a detector generates a pulse after it already has detected a photon, is minimal, and dead time is non excitant. Our patented high-end microchannel plate technology offers a high dynamic range, an unmatched collection efficiency (CE) and excellent temporal characteristics.
Vacuum Tube Detector Working Principle
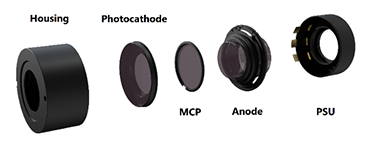
A vacuum tube photon detector is a type of detector that uses a vacuum tube to detect photons. It works on the principle of the photoelectric effect, which is the emission of electrons from a material when it absorbs electromagnetic radiation, such as light.
The vacuum tube photon detector consists of a vacuum tube, which is a sealed container that contains a vacuum. Inside the vacuum there is a cathode, an MCP and an anode separated by a small gap. The cathode is a metal surface that emits electrons when it is struck by photons. The MCP multiplies the generated photoelectrons, and the anode is also a metal surface that collects the emitted electrons.
When photons strike the photocathode, they are absorbed by the metal surface, causing electrons to be emitted. These emitted photoelectrons are then accelerated towards the MCP by an applied voltage, which generates a current that is proportional to the number of detected photons. The vacuum inside the tube ensured that the electrons do not collide with any gas molecules, which could otherwise interfere with the detection process.
Single photon counting using an MCP-PMT
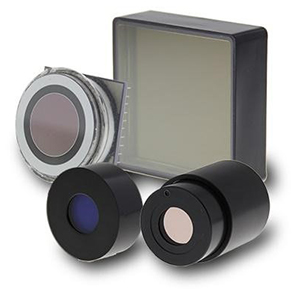
MCP-PMTs are commonly used in high-end LIDAR applications, various fields such as medical imaging, nuclear physics, and astronomy. In these fields of research, counting single photons is essential for accurate detection and measurement. Fast MCP-PMTs can detect very low levels of light and produce a high gain output signal, making them an ideal choice for detecting individual photons of light.
MCP-PMTs consist of a window + photocathode, a stack of micro-channel plates (MCPs), and an anode.
In single photon counting applications, MCP-PMTs operate in the following way:
- A photon enters the MCP-PMT and interacts with the photocathode, causing the emission of an electron.
- The emitted electron is accelerated and multiplied as it passes through the stack of micro-channel plates (MCPs) which are essentially thin plates with many microscopic channels.
- The multiplied electrons are then collected by the anode, which produces an output signal that can be detected and analyzed.
MCP-PMTs are particularly suited to single photon counting applications because they have a very high quantum efficiency, which means they can convert a high percentage of incident photons into an electron signal. They also have a fast response time, can detect very low levels of light and generate an extreme low number of dark counts.
Single photon Imaging using an Image Intensifier Tube (IIT)
The use of IITs in single photon imaging applications can greatly improve the sensitivity of the system, allowing for the detection and imaging of individual photons. In addition to their use in biological imaging, quantum imaging, and astronomy, IITs are also used in a variety of other applications, including night vision, military imaging, and industrial inspection.
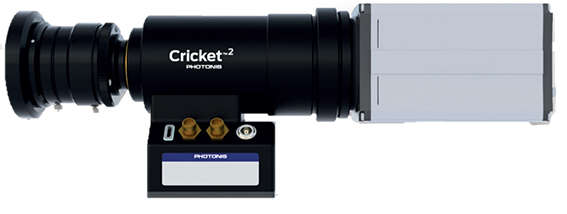
The basic principle of single photon imaging using an IIT is as follows:
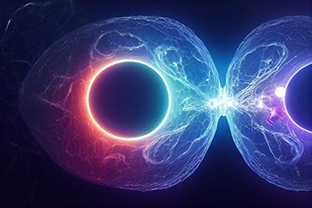
- A photon enters the IIT through the input window and strikes a photocathode, which is typically made of a material such as cesium or potassium.
- The photon causes the photocathode to emit electrons, which are then accelerated towards a microchannel plate (MCP) by an electric field.
- The electrons passing through the MCP cause a cascade of secondary electrons, resulting in a significant amplification of the electron signal.
- The amplified electron signal is then accelerated towards a phosphor screen, which emits visible light when struck by electrons.
- A sensitive camera, such as an EMCCD or sCMOS camera, is used to capture the light emitted by the phosphor screen and produce an image.
The Cricket™² contains an Image intensifier tube (IIT) which are often used in single photon imaging applications to amplify the signal from individual photons and produce a measurable output signal. In single photon imaging, the goal is to detect and image single photons, which can be very difficult due to the extremely low levels of light involved. IITs can help to overcome this challenge by providing a high gain amplification of the photon signal, making it possible to detect and image single photons.
-------------------------------------------------------------------------------------------------
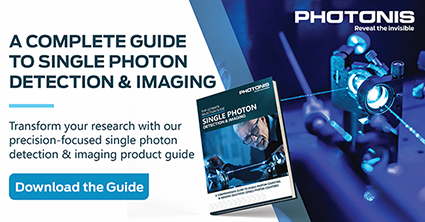
-------------------------------------------------------------------------------------------------
Single Photon Imaging and Counting Using a TPX3 Chip
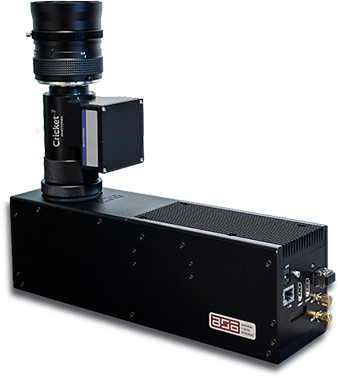
The Mantis3 combines the TPX3CAM with a Cricket™² thereby creating a single photon sensitive, state-of-the-art imaging system. The Mantis3 was specially developed for use in high-resolution imaging and spectroscopy applications and various quantum imaging applications, including single-photon detection, quantum key distribution, quantum entanglement, and quantum teleportation.
The Timepix3 chip used in the Mantis3 is a hybrid-pixel detector containing 256 x 256 pixels, a high time resolution of less than 1 nanosecond and a high spatial resolution of 55 micrometers, which makes it ideal for quantum imaging applications. The Mantis3 also features a high-speed readout and can operate at high frame rates of up to 1,000 frames per second.
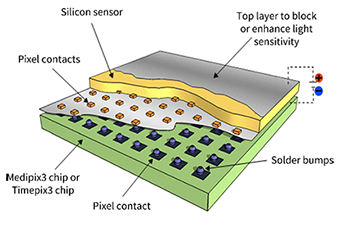
One of the key features of the Mantis3 is its ability to perform simultaneous energy and time-resolved imaging. This is achieved through the use of a Time-over-Threshold (ToT) technique, which allows the camera to measure the energy of each detected particle or photon as well as its time of arrival. This enables the Mantis3 to produce high-resolution images and spectra of a wide range of samples, including biological tissues, materials, and subatomic particles.
The Mantis3 is also highly configurable, which makes it ideal for use in a wide range of imaging applications. It can be configured to detect particles or photons in different energy ranges, and it can also be programmed to detect specific patterns of particles or photons that are associated with certain phenomena.
Overall, the TPX3CAM is a powerful imaging system that is specifically designed for quantum applications. Its high time and spatial resolution, high-speed readout, real-time data analysis capabilities, and configurability make it an ideal tool for a wide range of quantum imaging applications.
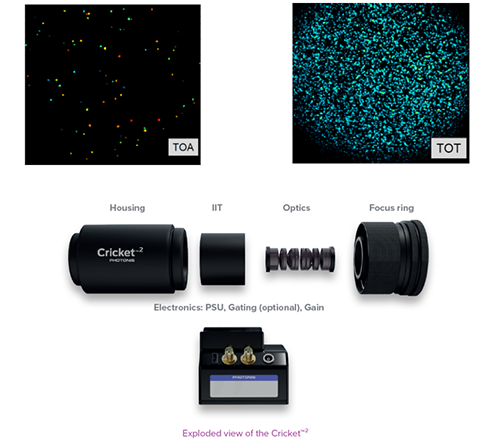
-------------------------------------------------------------------------------------------------
To learn more about Photonis' current single photon counting and imaging technology, visit the product page:
-------------------------------------------------------------------------------------------------
Frequently Asked Questions (FAQs)
How do Vacuum Tube-Based Detectors Work?
Vacuum tube-based Image Intensifier tubes consist of several essential components; a Photocathode, a Microchannel Plate (MCP) and an anode. These components work together to amplify input signal, creating a rich and dynamic output.
In the first step, existing ambient light passes through a photocathode, which converts the incoming photon signal into a photo-electron.
In the second step, photoelectrons are drawn by an electrical field into the MCP where they impinge multiple times on the inner walls and thereby multiply several thousands of times. In photon counting applications the multiplied electron signal is detected using an anode. In the instance of photon imaging applications, the anode converts the electron back into photons to produce an image.
What are the Main Benefits of Vacuum Tube-Based Detectors?
Vacuum tube-based single photon detectors offer several benefits for single photon detection applications compared to other technologies. Here are some of the main advantages:
- High sensitivity: Vacuum tube-based detectors are capable of detecting extremely low levels of light, down to the single photon level. This makes them well-suited for applications that require high sensitivity, such as quantum optics, fluorescence spectroscopy, and low-light imaging.
- Wide spectral range: Vacuum tube detectors have a wide spectral response range, spanning from ultraviolet (UV) to near-infrared (NIR) wavelengths. This versatility allows them to be used in a broad range of applications across different scientific disciplines.
- Fast response time: MCP-PMTs have fast response times, typically in the sub-nanosecond range. This enables them to accurately capture fast events or rapidly changing light signals, making them suitable for time-resolved measurements and applications requiring high temporal resolution.
- Large active area: MCP-PMTs have relatively large active areas compared to other single photon detectors. This makes them capable of detecting photons over a larger spatial area, which is advantageous for applications such as imaging and light detection in broad fields of view.
- Low noise: Vacuum tube-based detectors exhibit low noise characteristics, allowing for excellent signal-to-noise ratios. This is especially important for detecting weak light signals and enhancing the accuracy of measurements.
- High gain: MCP-PMTs provide high gain amplification due to their electron multiplication stages. Each photon that enters the detector can generate a cascade of electrons, resulting in a significantly amplified output signal. This high gain makes it easier to detect and measure single photons with improved signal quality.
- Versatility: Vacuum tube-based detectors can be used in a wide range of experimental setups and configurations, including single photon counting, photon correlation spectroscopy, fluorescence lifetime measurements, and many others. They are adaptable to different experimental requirements and can be integrated into various optical systems.
What are the Main Challenges in Single Photon Detection?
The main challenges in single photon detection include:
- Detection efficiency: The detection efficiency refers to the probability of a photon being detected by the detector. Achieving high detection efficiency is crucial in single photon detection applications. The efficiency depends on factors such as the detector technology, photon wavelength, and optical coupling efficiency. Maximizing detection efficiency is essential for capturing the highest possible number of photons.
- Timing resolution: Many applications involving single photon detection require precise timing information, such as in time-correlated single photon counting (TCSPC) or quantum cryptography. Achieving high timing resolution is challenging, as it requires fast electronics and detectors with short response times to accurately capture the arrival times of individual photons.
- Spatial resolution
- Spectral resolution
- Environmental and operating conditions
- Integration and scalability: In some applications, there is a need for miniaturized or integrated single photon detectors. Challenges arise in developing compact, robust, and efficient detector designs that can be integrated into complex systems or small-scale devices while maintaining high performance.
What Impacts the Detection Efficiency of Single Photon Detectors?
Quantum Efficiency (QE) is a key objective in the development of single photon detectors, as it directly impacts the overall performance of the device.
What are the Limitations of Current Single Photon Detection Technologies?
Current single photon detection technologies often struggle to achieve high performance across all relevant metrics, such as sensitivity, timing resolution, spatial resolution, and spectral resolution, without compromising on other aspects of detector performance.
What are the Potential Applications of Single Photon Detection in the Future?
Single photon detection has potential applications in a wide range of fields, including quantum communication and computing, biomedical imaging, LIDAR, astronomy, and remote sensing.
How do Researchers Plan to Overcome these Technical Challenges?
Researchers are exploring novel materials, device architectures, and fabrication techniques to address the technical challenges in single photon detection. This includes the development of new materials, such as 2D materials or perovskites, improved detector designs, advanced signal processing algorithms, and innovative cooling and shielding techniques. By pushing the boundaries of what is possible in single photon detection, researchers aim to unlock the full potential of this groundbreaking technology for a wide range of scientific and industrial applications.